Chinese researchers find bat virus enters human cells via same pathway as COVID
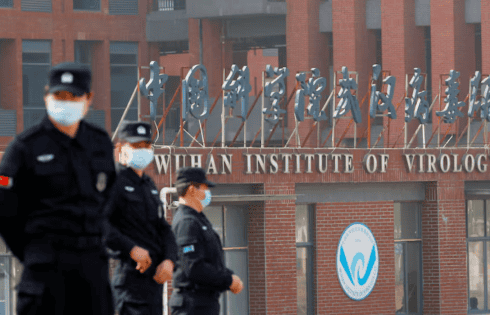
In a significant development for pancreatic cancer treatment, a phase 1 clinical trial has highlighted the potential of personalized mRNA vaccines to treat the deadly disease.
This research comes at a critical time, as pancreatic cancer is known for its grim prognosis, with less than 13% of patients surviving beyond five years.
The disease is often diagnosed at advanced stages due to a lack of early symptoms and routine screening.
Pancreatic cancer is particularly challenging because around 90% of cases are found when they are already advanced.
The nature of this cancer often allows it to spread to other parts of the body before it can be effectively treated.
Traditional methods like surgery, chemotherapy, and radiation are limited in their ability to cure the disease, which has led researchers to seek innovative therapies.
Dr. Vinod Balachandran, who leads the Olayan Center for Cancer Vaccines at Memorial Sloan Kettering Cancer Center, expressed the urgent need for new options.
“Despite advancements in treatments for other cancers, pancreatic cancer remains largely resistant, with a survival rate that has stagnated around 10% even following the best available therapies,” he noted.
Before the COVID-19 pandemic popularized mRNA technology, researchers already explored its potential for cancer treatment.
For an mRNA vaccine to be effective, it must generate a strong response from T cells, a type of immune cell that combats infections and diseases. These T cells must remain active over time to fight cancer cells.
While training T cells to attack viruses is comparatively straightforward, teaching them to target cells the body has created is considerably more complex.
The challenge is particularly pronounced in pancreatic cancer. For a vaccine to successfully prompt the immune system to identify tumor cells, there must be unique targets present on those cells.
Cancer cells develop from mutations, and these mutations can serve as targets. However, pancreatic tumors generally have fewer mutations available, leading to skepticism about the effectiveness of mRNA vaccines for this cancer type.
The recent trial aimed to test this assumption by exploring the efficacy of personalized mRNA vaccines in a group with operable pancreatic cancer.
This was a follow-up study to an initial trial conducted earlier in 2023.
Phase 1 trials are designed to assess safety and initial effectiveness, and this new study included 16 patients whose tumors could be surgically removed—a rare situation, as only about 20% of pancreatic cancers are operable.
In addition to surgical treatment, these participants were given personalized mRNA vaccines tailored to their tumor profiles.
The researchers monitored the patients for up to four years after tumor removal, assessing the vaccine’s ability to stimulate an immune response.
Results showed that half of the participants—eight out of 16—responded positively to the vaccine, producing T cells capable of targeting their tumors.
A few years ago, a new NGS platform being announced at the Advances in Genome Biology and Technology (AGBT) meeting would not have been a surprise; multiple new instruments entered the NGS arena over the span of a few years. In turn, the genomics community has grown used to teams touting their “game-changing” platforms, with promises of either lower cost, longer reads, higher accuracy, more throughput (or all of the above!) But those days had settled down. Or so we thought.
This week, Roche made it feel like 2022 again. Just days before the AGBT meeting kicks off in Marco Island, FL, the company hosted a much-anticipated webinar to offer a technical introduction to their sequencing expansion technology (SBX): a coming together of two companies that Roche had previously acquired—Stratos Genomics and Genia Technologies.
Why now for the technology unveiling? “It just felt like this was the right timing based on where we were, and our comfort with where we are with the technology which we’re very excited about,” noted Mark Kokoris, vice president, head of SBX technology RS.
Kokoris, who co-founded Stratos Genomics in 2007 and served as the company’s CEO until the acquisition, invented SBX technology. The webinar was designed to be “a heavy dive in on the technical,” he told GEN, with less focus on the timeline of the instrument launch and more focus on “the technology, what we’re putting forward, and what we’re seeing.”
“Our approach to efficiently sequencing DNA is to not sequence DNA,” Kokoris told GEN, chuckling. Instead, he explained, they created and innovated a biochemical conversion process to change DNA into an expanded surrogate molecule with the idea to rescale the signal-to-noise problem. He brought the idea to his friend Bob McRuer, Stratos’ former CTO, almost 20 years ago, who loved it because it simplified measurement. Then, Kokoris had to figure out how to make the chemistry come together without a roadmap.
Nothing existed, he remembers, to explain how to build a cleavable X-NTP (one of the keys to the technology). When you see the structure of an X-NTP, Kokoris asserted, you probably think, this is crazy! “And I knew it was going to require innovation in protein engineering, molecular engineering, and developing chemistries that didn’t exist. And there was a bunch of other stuff that we didn’t realize at the time that we had to innovate.”
SBX creates a surrogate molecule called an Xpandomer (which is 50 times longer than target DNA) and encodes the DNA sequence information in large, high signal-to-noise reporters. The backbone of the Xpandomer is X-NTPs, which are linked along a target DNA template. The DNA sequence is represented in the X-NTP sequence. The four X-NTP types have a tether that is linked between the base and the alpha phosphate. In the process, the DNA template is degraded and the backbone expands, becoming an Xpandomer which is pulled through a nanopore. This is performed in millions of wells on a CMOS-based sensor.
“When we got to the other side of [building the technology] it was exactly what we had thought. We had solved that single molecule signal to noise. And now that we have transitioned that to a large, 8 million array. It’s bonkers.”
Roche is not a newcomer to the sequencing space: the company bought Jonathan Rothberg’s 454 in 2007 for $140 million. 454 had just announced the completion of Jim Watson’s genome (in May 2007) and published in Nature the following the year. But it was evident that 454’s platform could not get the WGS price down significantly, while competition from Illumina and others was rapidly intensifying (PacBio launched at AGBT in 2008). The writing was on the wall when Nature published three back-to-back landmark NGS papers in November 2008—the first African genome, the first Asian genome, and the first cancer genome—all featuring the Illumina platform.
Roche shut down the 454 program in 2013. Meanwhile, Roche had taken a shine to Genia Technologies, the developer of a single molecule, semiconductor-based, DNA sequencing platform using nanopore technology. Roche eventually bought Genia for $350M in 2014.
At this point, a new NGS technology has to offer something new or different to users to get noticed. What are the advantages of SBX? Is it read length, cost, accuracy? According to Kokoris, the cost, scale, throughput, and accuracy have all been considered. (The data from the webinar suggested that read accuracy and speed are strengths of the system.) But the one thing that was always the focus—even back when McRuer and he would meet at Starbucks on First Avenue (in Seattle) for six-hour sessions back in 2007—was flexible operation. That was at the top of mind then and “hasn’t changed one bit in 18 years,” noted Kokoris.
“We envisioned a platform that can sequence up and down the throughput spectrum with impunity,” Kokoris explained. “In other words, one system where someone could do four minutes, 40 minutes, or four hours depending on your throughput. That’s what I wanted on one system. And that is why we did single molecule.”
The technology will be released as research use only, but Kokoris noted that there are future ambitions to take it to the clinical setting. This year will be early access, with the plan to commercialize in 2026. No other details on pricing or sample prep were offered at this time.
At the end of the webinar, Kokoris thanked his team and many others. And he thanked those who believed in this when “it seemed like a crazy idea.” Although Kokoris was referring to the technology, it might also seem crazy to some to launch a new NGS technology into an already extremely crowded market. Time will tell. But the SBX announcement has succeeded in creating a buzz that the NGS community hasn’t experienced for a few years. And in the words of Peter Diamandis, MD: “The day before something is a breakthrough, it’s a crazy idea.”
A new study suggests a potential molecular strategy for treating fragile X syndrome, an inherited neurodevelopmental disorder that causes autism spectrum disorder and intellectual disability. This work shows that enhancing the function of the GluN2b subunit of the N-methyl-D-aspartate (NMDA) receptor signaling pathway can correct key neural dysfunctions in a mouse model of fragile X syndrome.
The research, titled “Non-ionotropic signaling through the NMDA receptor GluN2B carboxy-terminal domain drives dendritic spine plasticity and reverses fragile X phenotypes,” was published in Cell Reports.
Led by Mark Bear, PhD, at MIT’s Picower Institute for Learning and Memory, the study builds on previous work by this group exploring the role of NMDA receptors in regulating synaptic plasticity. The group studies synaptic plasticity and has a history of exploring the molecular basis of fragile X syndrome, and a related disorder, tuberous sclerosis (Tsc).
“We ended up here by accident at the end of the last century, following up on studies of the basic neurobiology of synaptic plasticity. One of our discoveries suggested the possibility that we could reverse aspects of fragile X syndrome, and we have stuck with it for 25 years,” Bear shared with GEN.
Fragile X is characterized by excessive protein synthesis, which leads to synaptic dysfunction and predisposition to seizures, while Tsc involves reduced protein synthesis. In fact, crossbreeding mouse models of both conditions results in healthy offspring, with the protein expression levels balancing each other.
NMDA receptors play a critical role in synaptic plasticity, where calcium ions flow through the receptor and contribute to long-term depression. More recent work from the group identified a signaling pathway for NMDA that was independent of ion flow.
The team hypothesized that two subunits of NMDA, GluN2A and GluN2B, have separate functions contributing to the two functional pathways, with GluN2A contributing to synaptic function via ion flow, while GluN2B modifies protein synthesis through the non-ionotropic mechanism.
To test these hypotheses, the team used the shrinkage and enlargement of dendritic spines as a physical marker for synaptic plasticity in response to modifications in NMDA function. Knockout experiments showed that loss of either GluN2A or GluN2B disrupted long-term depression, a consequence of ionotropic signaling. Knocking out GluN2B eliminated spine shrinkage, a hallmark of synaptic plasticity through non-ionotropic signaling.
Bear commented that by using this method, the team “discovered a novel approach to rebalance altered protein synthesis regulation in these diseases, one that had not been known before.”
The MIT researchers genetically engineered mice in which the carboxy-terminal domain (CTD) of GluN2B was swapped with that of GluN2A. They found that disrupting GluN2B’s CTD eliminated its ability to regulate spine size and increased bulk protein synthesis, mirroring what is seen in fragile X syndrome. Conversely, enhancing GluN2B signaling reduced protein synthesis to normal levels, similar to what is observed in Tsc models.
They then tested whether increasing GluN2B signaling could counteract fragile X-like phenotypes. Fragile X model mice were treated with Glyx-13, which selectively binds to GluN2B. Treatment normalized protein synthesis and reduced seizure susceptibility in fragile X model mice, suggesting that targeting GluN2B could represent a novel therapeutic approach for fragile X syndrome.
“These findings suggest that non-ionotropic NMDAR signaling through GluN2B may represent a novel therapeutic target for the treatment of fragile X and related causes of intellectual disability and autism,” the authors wrote.
By putting together data collected over decades, the MIT researchers identified a deeper understanding of a signaling pathway with broad implications for neurological disorders. Bear pointed out to GEN that “fragile X and TSC are monogenic causes of autism spectrum disorder.” He continued, “We expect that insights gained by studying these diseases will be broadly applicable.”
“One of the things I find most satisfying about this study is that the pieces of the puzzle fit so nicely into what had come before,” said Bear.
The international Healthy Crops consortium has developed an innovative strategy to combat the disease bacterial blight (for short: BB) in rice using genome editing technology. If approved for use by farmers in Kenya, the BB-resistant rice varieties are expected to reduce yield losses associated with the disease in the affected rice growing regions and increase productivity. The work is a collaboration between Kenya Agricultural and Livestock Research Organization (KALRO) and Heinrich Heine University Düsseldorf (HHU).
Rice production is of central importance for food security and economic development in many countries, in particular in low- and medium-income countries. Rice is the second most important staple food in East Africa, with 1.8 million tonnes consumed every year in the countries of the East African Community (for short: EAC).
In 2019, members of the Healthy Crops team identified an outbreak of BB in Tanzania caused by invasive Asian variants of the bacterium Xanthomonas oryzae pv. oryzae (Xoo). The bacterium is spreading rapidly and causing estimated yield losses of 13–20%.
Dr. Emily Gichuhi from KALRO explains, “Due to climate change, incidences of rice diseases including BB have been on the rise in Kenyan rice growing areas. This has increased the cost of production among rice farmers, thereby reducing their returns.”
Dr. Daigo Makihara and Dr. Moto Ashikari from Nagoya University (NU) in Japan, researchers from the Wonder Rice Initiative for Food Security and Health (WISH), are working closely with Dr. Gichuhi and her team to develop new African rice varieties. Dr. Makihara explains, “As a result of the international spread of different crop plant varieties, we are increasingly finding ourselves confronted with outbreaks of plant diseases in regions where they have not previously played a role.”
The starting point for the researchers from Healthy Crops is the nutrient supply of the bacteria. The Xoo bacteria possess a set of “keys” which can open the “pantry” of the plants: When the bacterium injects one of these “key” proteins into rice cells, it leads to increased production of a transporter, which releases sugar in the neighborhood of the bacteria. This sugar serves as nutrition and is essential for the multiplication and virulence of the bacteria. However, when the bacteria utilize the sugar, there is none left for the plant, which ultimately dies as a result.
The research team has succeeded in changing the “locks” via genome editing, making the plants resistant to all known Xoo strains currently prevalent in Asia and Africa.
Professor Bing Yang, University of Missouri, who developed the editing approach, states: “The combination of two different sets of enzymes for editing enabled us to develop a robust resistance.”
The import of these edited elite rice varieties has been made possible due to the availability of genome editing guidelines developed by the National Biosafety Authority (NBA) of Kenya and published in 2022.
Dr. Marcel Buchholzer, coordinator of the Healthy Crops project at HHU, explains, “It is now possible to evaluate these rice lines, developed using advanced biotechnology methods at HHU, in Kenya.”
Professor Dr. Wolf B. Frommer, spokesperson for the project at HHU explains, “This project aims to protect smallholder farmers from crop yield losses through knowledge-based approaches to fighting plant diseases.”
Northwestern University researchers have identified structural features in engineered cell receptors that correlate with variations in receptor function.
Computational protein structure prediction tools were used to analyze a library of synthetic receptors, revealing that specific structural attributes such as ectodomain (ECD) distance and transmembrane domain (TMD) interactions are associated with receptor performance.
Engineered cell therapies rely on synthetic receptors to transduce external signals into intracellular responses. The precise relationship between receptor structure and function remains poorly understood. Advances in protein structure prediction tools, such as AlphaFold and ColabFold, have enabled the modeling of complex proteins, including single-pass transmembrane receptors.
In the study, “Exploring Structure-Function Relationships in Engineered Receptor Performance Using Computational Structure Prediction,” published in GEN Biotechnology, researchers integrated predicted protein conformations with experimental performance metrics across four engineered receptor families, examining whether certain molecular features correlated with functional output.
Researchers analyzed a library of engineered receptors derived from natural cytokine receptors, focusing on Modular Extracellular Sensor Architecture (MESA) receptors. MESA receptors use ligand-induced dimerization to activate a split Tobacco Etch Virus Protease (TEVp), which releases a transcription factor to drive gene expression.
ColabFold was used to generate structural models of receptor ECDs in complex with their ligands, while PREDDIMER was employed to model TMD interactions.
Structural features were quantified, including ECD distance, ECD contacts, TMD distance, TMD contacts, TMD crossing angle, and TMD exit angle. Receptor performance was assessed based on on-state reporter expression (OS) and fold induction (FI) in experimental data.
Substantial variation in receptor performance was explained by structural features. Shorter ECD and TMD distances correlated with higher OS, while greater ECD and TMD contacts were also associated with improved performance. TMD exit angle had a significant relationship with receptor function in certain receptor families, particularly in tumor necrosis factor (TNF) MESA.
Structural models explain less variation in performance for heterotetrameric receptors, such as interleukin-10 (IL-10) MESA and transforming growth factor beta (TGFb) MESA. This suggests that additional factors, such as higher-order receptor complex formation, may contribute more to the performance of these receptors.
To complement structural analyses, the researchers evaluated categorical variables representing domain choices within receptor chains. One-hot encoding of receptor components revealed that VEGF MESA receptors containing a CD28 TMD in the C-terminal chain and a VEGFR1 ECD exhibited higher OS. The reduced categorical model explained 57% of OS variation, closely aligning with the structural model’s performance.
A combined model integrating both structural and categorical features demonstrated superior predictive power, explaining 79% of OS variation. This improvement indicates that while structural and categorical models share some information, they capture distinct determinants of receptor performance.
The findings present actionable hypotheses for synthetic receptor engineering. For instance, modifying receptor linkers to enhance ECD contacts or selecting TMD pairs that favor closer C-terminal proximities could improve receptor function.
A notable challenge in this work was modeling the entire single-pass transmembrane receptor,including the natural ectodomain (ECD), the transmembrane domain (TMD), and the split TEVp intracellular domains (NTEVp or CTEVp) using ColabFold.
Specifically, the tool struggled with aligning the shorter TEVp regions within long receptor sequences. During the multiple sequence alignment (MSA) search step, the sizable ECD and native TMD often dominated the alignment results, causing ColabFold to drop or poorly cover the TEVp segments.
As a result, the predicted structures sometimes failed to show a properly folded NTEVp or CTEVp, yielding low-confidence scores (pLDDT) in those intracellular portions.
Shorter ectodomains (e.g., IL-10 receptors) were more likely to retain coverage for the TEVp domains, allowing a few full-length models to converge on structures where the NTEVp and CTEVp were reconstituted.
Because of these alignment issues, the study opted for a modular approach, modeling ECD–ligand complexes separately with ColabFold and then using PREDDIMER to capture TMD–TMD interactions.
While this strategy prevented direct visualization of how NTEVp and CTEVp might orient themselves under membrane constraints, it ensured higher confidence in the predicted extracellular and transmembrane regions. The trade-off is that intermolecular effects were not fully captured.
A vaccine for pancreatic cancer could serve as a promising new therapy.
At Memorial Sloan Kettering Cancer Center (MSK) in New York, an experimental approach to treating the cancer with a messenger RNA (mRNA)-based therapeutic vaccine “continues to show potential” in reducing the risk of the disease returning after surgery, according to a press release.
Results from the phase 1 clinical trial, published in the journal Nature, revealed that the vaccine triggered an immune response in a small patient group.
This immune-cell activation persisted for nearly four years after treatment, the release stated.
Patients who received the vaccine and showed an immune system response were more likely to remain cancer-free by their three-year follow-up.
Vinod Balachandran, MD, principal investigator of the trial and senior study author at MSK, stated his optimism about the efficacy of this treatment.
“We find that with RNA vaccine technology, we can teach the immune system to recognize pancreatic cancer, and this immune response could potentially last for many years,” he said.
“The ability to trigger a robust, long-lasting immune response is a requisite feature for any cancer vaccine.”
As pancreatic cancer is “difficult to treat,” Balachandran shared his surprise that the immune system responded in some patients.
“We are encouraged to see that our approach can teach the immune system to recognize pancreatic cancer, that the immune response is potent and that it may potentially impact patients,” he said.
“The ability to trigger a robust, long-lasting immune response is a requisite feature for any cancer vaccine.”
“Although the findings are promising, these vaccines are still in the early stages of testing,” Balachandran said.
The team is planning a larger study “to further test these therapeutic cancer vaccines in patients with surgically removable pancreatic cancer.”
Of the 16 participants, the vaccine stimulated an immune response in half of them. The cancer vaccine was personalized for each participant to target specific proteins found in their form of the disease.
The patients also received an immunotherapy drug (atezolizumab) and standard-of-care chemotherapy after receiving surgery.
Therapeutic cancer vaccines like this one, referred to as autogene cevumeran, are meant to treat the cancer instead of preventing it, by training the immune system to “recognize cancer cells as foreign,” according to the researchers.
The vaccine is meant to target early stages of cancer that have not spread, where tumors can be surgically removed, to “help delay or prevent recurrence.”
Balachandran noted that this approach could potentially be applied to other forms of cancer as well.
“If you can do this in pancreatic cancer, theoretically you may be able to develop therapeutic vaccines for other cancer types.”
“For patients with pancreatic cancer, our latest results continue to support the approach of using personalized mRNA vaccines to target neoantigens in each patient’s tumor,” he said.
“If you can do this in pancreatic cancer, theoretically you may be able to develop therapeutic vaccines for other cancer types.”
Pancreatic cancer remains one of the deadliest cancers, as only about 13% of patients are alive five years following diagnosis, according to MSK and the American Cancer Society.
Common therapies like chemotherapy, radiation and immunotherapies are “largely ineffective” against pancreatic cancer, MSK stated, which makes new therapies “urgently needed.”
All biological function is dependent on how different proteins interact with each other. Protein-protein interactions facilitate everything from transcribing DNA and controlling cell division to higher-level functions in complex organisms.
Much remains unclear, however, about how these functions are orchestrated on the molecular level, and how proteins interact with each other—either with other proteins or with copies of themselves.
Recent findings have revealed that small protein fragments have a lot of functional potential. Even though they are incomplete pieces, short stretches of amino acids can still bind to interfaces of a target protein, recapitulating native interactions. Through this process, they can alter that protein’s function or disrupt its interactions with other proteins.
Protein fragments could therefore empower both basic research on protein interactions and cellular processes, and could potentially have therapeutic applications.
Recently published in Proceedings of the National Academy of Sciences, a new method developed in the Massachusetts Institute of Technology Department of Biology builds on existing artificial intelligence models to computationally predict protein fragments that can bind to and inhibit full-length proteins in E. coli. Theoretically, this tool could lead to genetically encodable inhibitors against any protein.
The work was done in the lab of associate professor of biology and Howard Hughes Medical Institute investigator Gene-Wei Li in collaboration with the lab of Jay A. Stein (1968) Professor of Biology, professor of biological engineering, and department head Amy Keating.
The program, called FragFold, leverages AlphaFold, an AI model that has led to phenomenal advancements in biology in recent years due to its ability to predict protein folding and protein interactions.
The goal of the project was to predict fragment inhibitors, which is a novel application of AlphaFold. The researchers on this project confirmed experimentally that more than half of FragFold’s predictions for binding or inhibition were accurate, even when researchers had no previous structural data on the mechanisms of those interactions.
“Our results suggest that this is a generalizable approach to find binding modes that are likely to inhibit protein function, including for novel protein targets, and you can use these predictions as a starting point for further experiments,” says co-first and corresponding author Andrew Savinov, a postdoc in the Li Lab. “We can really apply this to proteins without known functions, without known interactions, without even known structures, and we can put some credence in these models we’re developing.”
One example is FtsZ, a protein that is key for cell division. It is well-studied but contains a region that is intrinsically disordered, and therefore, especially challenging to study. Disordered proteins are dynamic, and their functional interactions are very likely fleeting—occurring so briefly that current structural biology tools can’t capture a single structure or interaction.
The researchers leveraged FragFold to explore the activity of fragments of FtsZ, including fragments of the intrinsically disordered region, to identify several new binding interactions with various proteins. This leap in understanding confirms and expands upon previous experiments measuring FtsZ’s biological activity.
This progress is significant in part because it was made without solving the disordered region’s structure, and because it exhibits the potential power of FragFold.
“This is one example of how AlphaFold is fundamentally changing how we can study molecular and cell biology,” Keating says. “Creative applications of AI methods, such as our work on FragFold, open up unexpected capabilities and new research directions.”
The researchers accomplished these predictions by computationally fragmenting each protein and then modeling how those fragments would bind to interaction partners they thought were relevant.
They compared the maps of predicted binding across the entire sequence to the effects of those same fragments in living cells, determined using high-throughput experimental measurements in which millions of cells each produce one type of protein fragment.
AlphaFold uses co-evolutionary information to predict folding, and typically evaluates the evolutionary history of proteins using something called multiple sequence alignments (MSAs) for every single prediction run. The MSAs are critical, but are a bottleneck for large-scale predictions—they can take a prohibitive amount of time and computational power.
For FragFold, the researchers instead pre-calculated the MSA for a full-length protein once, and used that result to guide the predictions for each fragment of that full-length protein.
Savinov, together with Keating Lab alumnus Sebastian Swanson, Ph.D., predicted inhibitory fragments of a diverse set of proteins in addition to FtsZ. Among the interactions they explored was a complex between lipopolysaccharide transport proteins LptF and LptG. A protein fragment of LptG inhibited this interaction, presumably disrupting the delivery of lipopolysaccharide, which is a crucial component of the E. coli outer cell membrane essential for cellular fitness.
“The big surprise was that we can predict binding with such high accuracy and, in fact, often predict binding that corresponds to inhibition,” Savinov says. “For every protein we’ve looked at, we’ve been able to find inhibitors.”
The researchers initially focused on protein fragments as inhibitors because whether a fragment could block an essential function in cells is a relatively simple outcome to measure systematically. Looking forward, Savinov is also interested in exploring fragment function outside inhibition, such as fragments that can stabilize the protein they bind to, enhance or alter its function, or trigger protein degradation.
This research is a starting point for developing a systemic understanding of cellular design principles, and what elements deep-learning models may be drawing on to make accurate predictions.
“There’s a broader, further-reaching goal that we’re building towards,” Savinov says. “Now that we can predict them, can we use the data we have from predictions and experiments to pull out the salient features to figure out what AlphaFold has actually learned about what makes a good inhibitor?”
Savinov and collaborators also delved further into how protein fragments bind, exploring other protein interactions and mutating specific residues to see how those interactions change how the fragment interacts with its target.
Experimentally examining the behavior of thousands of mutated fragments within cells, an approach known as deep mutational scanning, revealed key amino acids that are responsible for inhibition. In some cases, the mutated fragments were even more potent inhibitors than their natural, full-length sequences.
“Unlike previous methods, we are not limited to identifying fragments in experimental structural data,” says Swanson. “The core strength of this work is the interplay between high-throughput experimental inhibition data and the predicted structural models: the experimental data guide us towards the fragments that are particularly interesting, while the structural models predicted by FragFold provide a specific, testable hypothesis for how the fragments function on a molecular level.”
Savinov is excited about the future of this approach and its myriad applications.
“By creating compact, genetically encodable binders, FragFold opens a wide range of possibilities to manipulate protein function,” Li agrees. “We can imagine delivering functionalized fragments that can modify native proteins, change their subcellular localization, and even reprogram them to create new tools for studying cell biology and treating diseases.”
One of nature’s most disliked creatures may very well unlock a breakthrough in disaster response. A multidisciplinary Purdue University research team is recreating mosquito antennae to better study their sensitivity to vibrations. Should the research prove fruitful, it could lead to improvements in monitoring and detecting natural disasters such as earthquakes and tsunamis.
Research groups under Purdue professors Pablo Zavattieri and Ximena Bernal conducted this work, which is published in the journal Acta Biomaterialia.
“We’re still in the early stages but we’re pretty optimistic that we’ll at least learn a great deal,” said Zavattieri, the Jerry M. and Lynda T. Engelhardt Professor of Civil Engineering in Purdue’s College of Engineering. “Taking inspiration from nature and using it to advance scientific research has been a core feature of engineering since the very beginning.”
Despite lacking traditional ears, mosquitoes rely on their antennae to navigate the auditory landscape, homing in on crucial sounds amid the background noise of their own wingbeats.
Through analysis of the mosquitoes’ antennal features—particularly the arrangement and morphology of sensory hairs—civil and construction engineering Ph.D. candidate and team researcher Phani Saketh Dasika said they have already gained profound insights into how these adaptations enhance the auditory sensitivity and selective response to environmental cues.
The team’s findings have provided key information for determining whether a mosquito’s antennae could inform the design of acoustic sensors.
“By modeling and contrasting the response of the antennae of species of mosquito using sound for different purposes, hearing mates or eavesdropping on frogs, we were able to tease apart features modulating hearing sensitivity and tuning,” said Bernal, a professor of biological sciences in Purdue’s College of Science. “Understanding how these structures work is the first step toward developing acoustic sensors inspired by their sensitive antennae.”
In terms of societal impact, insights from mosquito antennae could also inform the development of smart noise-canceling materials, Zavattieri said. These materials, potentially incorporating microfluidic channels or tunable metamaterials, could be used to create soundproofing panels for buildings, noise-canceling headphones or even acoustic cloaking devices.
As part of their effort to answer a decades-old biological question about how the hepatitis B virus (HBV) can establish an infection in liver cells, researchers led by teams at Memorial Sloan Kettering Cancer Center (MSK), Weill Cornell Medicine, and the Rockefeller University have identified a vulnerability that may open the door to new HBV treatments.
The team successfully disrupted the virus’s ability to infect human liver cells in the laboratory using a chromatin-destabilizing molecule CBL137, which is already in clinical trials against cancer. The results lay the groundwork for animal model studies and potential drug development.
Headed by chemical biologist Yael David, PhD, at MSK, working with hepatologist and virologist Robert Schwartz, MD, PhD, at Weill Cornell Medicine and Viviana Risca, PhD, at the Rockefeller University, the researchers reported on their findings in Cell, in a paper titled, “A nucleosome switch primes hepatitis B virus infection.”
Chronic HBV infection is an incurable pathogen responsible for causing liver disease and hepatocellular carcinoma, the authors wrote. “Over 325 million people worldwide are chronically infected by hepatitis B virus … leading to almost one million deaths annually despite the availability of effective vaccines.” Chronic infection leaves patients at risk for advanced liver disease, and HBV is estimated to cause nearly half of all hepatocellular carcinoma cases, the team continued.
The newly reported research began with a chance meeting and a longstanding paradox. Schwartz, an associate professor of medicine in the Division of Gastroenterology and Hepatology at Weill Cornell Medicine, was introduced to David about six years ago at a retreat for Weill Cornell Physiology, Biophysics, and Systems Biology graduate school faculty, where they both hold appointments.
“On the surface, our research programs seem to have no overlap,” David said. “He studies hepatitis B, while my lab focuses on understanding how gene expression is regulated through a process called epigenetics. However, I was fascinated to discover that viruses like hepatitis B hijack epigenetic mechanisms, even using human DNA-packaging proteins to regulate their activity.”
Not long after, study first author Nicholas Prescott, PhD, then a doctoral student in the Tri-Institutional PhD Program in Chemical Biology, was preparing for a stint in the David Lab at MSK’s Sloan Kettering Institute. “His interest in epigenetic regulation in pathogens immediately made me consider HBV an ideal model system for him to explore,” David said.
At the heart of the mystery that intrigued the researchers lies a key viral gene that encodes for the HBV X protein (HBx). This protein is essential for HBV to establish a productive infection in host cells and the expression of its viral genes. However, the X gene itself is encoded within the viral genome.
“This raises a classic chicken-and-egg question that has puzzled scientists for decades,” David said. “How does the virus produce enough X protein to drive viral gene expression and establish infection?” The authors further commented “… HBx is absent from the virion and must be expressed de novo in freshly infected hepatocytes …”
Furthermore, the gene that encodes protein X is considered the virus’s oncogene—that is, the gene responsible for the disease’s progression toward cancer, Prescott added. That’s because protein X degrades proteins in the host that are involved with DNA repair. Not only does this keep the host from silencing protein X’s activity, but the infected cells are also more likely to accumulate DNA errors that build up over the years and decades, leading to the development of cancer.
“One of the main challenges with treating hepatitis B is that the existing treatments can stop the virus from making new copies of itself, but they don’t fully clear the virus from infected cells, allowing the virus to persist in the liver and maintain chronic infection,” noted Schwartz, whose lab contributed biological and clinical expertise in the virus, as well as the human liver cell models used in the study. “The current standard of care (long-term treatment with oral nucleos(t)ide analogs or short-term treatment with interferon-alpha injections) halts viral replication but falls short of eradicating cccDNA in infected hepatocytes, allowing the minichromosome to persist and sustain chronic infection,” the investigators stated. “Even with long-term antiviral treatment, basal levels of the HBV oncogenic X protein (HBx) remain in hepatocytes to promote genomic instability and disease progression.”
The hepatitis B vaccine is effective, but maintaining immunity often requires booster shots. Moreover, it doesn’t help people who are already infected. This happens, for example, due to transmission of the virus from mother to child, which is very common in developing countries. Access to vaccines and treatment is also more limited in some parts of Africa and Asia, where rates of infection are higher.
Digging into the mystery of protein X was a challenge, explained Prescott, who is now a postdoctoral fellow in the Laboratory of Chromosome and Cell Biology at the Rockefeller University. The existing tools weren’t capable of shedding light on what was happening in those critical early hours of an infection.
Early in infection, HBV establishes an independent “minichromosome” consisting of the viral covalently closed circular DNA (cccDNA) genome and host histones. But as the authors noted, “Despite extensive study, a significant knowledge gap remains regarding the role of viral chromatin status in establishing infection.”
The David Lab’s expertise is in how DNA gets packaged, read, and modified proved essential. For their newly reported research, the researchers successfully generated the HBV minichromosome for the first time, using their capabilities in reconstituting viral DNA in complex with human histones—the proteins that package and organize DNA. The research team determined that in order for protein X to get made, the hepatitis B virus’s DNA needs to get organized into DNA-histone complexes called nucleosomes. “… we generated recombinant, chromatinized cccDNA, allowing us to characterize its biophysical properties and to map nucleosome positioning on the minichromosome,” the scientists explained.
“This platform became a powerful tool not only to study the virus’s biochemistry but also to analyze, in detail, what happens in the critical first hours of an infection,” David said.
Nucleosomes are like beads on a string—the string is the viral DNA, and the beads are host-provided histone proteins, around which DNA gets wrapped; nucleosomes are the building blocks of chromatin, the material that makes up chromosomes.
It was this part of the project that tapped into the expertise of Risca at Rockefeller University. The Risca Lab studies the 3D architecture of the genome and how the packaging of DNA helps to control the transcription of genes. They had the tools and expertise to ensure that what the scientists were seeing in the new platform for studying the virus matched the reality of a human infection.
“Conventional wisdom says that packaging a gene’s DNA into nucleosomes would block or slow down the cell’s ability to read out that gene to make functional proteins, like protein X,” Risca commented. “But in complex organisms like humans and in the viruses that infect us, gene regulation is not always so straightforward. The presence and the positioning of nucleosomes on DNA can be important in directing cellular mechanisms to transcribe some genes. We found that to be the case for the HBV gene encoding protein X—the presence of nucleosomes on the viral genome is necessary for the transcription of RNA that gives rise to functional protein X.” The authors added. “Altogether, these data suggest a correlation between early cccDNA chromatinization and X transcription, which both occur during the first hours of infection.”
This discovery opens the door to understanding how the X gene is regulated and how HBV infection is established. Moreover, the researchers discovered a potential therapeutic opportunity. If it’s possible to disrupt the formation of these chromatin structures, then this could disrupt the virus’s ability to start and maintain an infection. “Given this link between chromatinization and transcription, we next hypothesized that disrupting chromatin assembly might inhibit viral transcription,” they wrote.
The team tested five small-molecule compounds known to impair chromatin formation. Only one, an anticancer drug candidate called CBL137, blocked production of protein X in liver cells.
Importantly, the compound worked in vitro at very low concentrations—many times smaller than participants in clinical trials for cancer were receiving, and using doses that only affected the virus, but not human cells. Their experimental results, they noted, “… demonstrate CBL137 as an effective inhibitor of HBV transcription and replication that may pose a potential therapeutic avenue to treat infections.”
David noted, “This made us very optimistic about the possibility of developing a treatment approach while preventing or limiting side effects. Moreover, if these results are confirmed through additional study, we are optimistic the approach could be used to treat chronic infections for the first time—and therefore could represent a potential cure.”
Additionally, CBL137 might prove similarly useful to target or study other chromatinized DNA viruses such as herpesviruses and papillomaviruses, the researchers noted. “Chromatin destabilization by CBL137 merits further investigation to test if it could prove similarly effective on other pathogens. Indeed, recent studies reported encouraging results for CBL137 as a latency reversing agent in HIV-1 infection and even a lead for drug discovery efforts against human African trypanosomiasis, underscoring its potential as a therapeutic against infectious diseases.
The project David pointed out, “…started from our fundamental interest in how the virus’s chromosomes might look and function and led to unexpected discoveries of how the viral infection is established in human cells.”
To further develop the team’s research toward a potential clinical trial, the next step would be to study the safety and effectiveness of CBL137 in animal models—though these are limited due to the narrow range of species HBV can infect, the researchers said.
All of the researchers stressed that the study wouldn’t have been possible without the close collaboration between the three institutions, which brought together the necessary expertise and technological resources—from MSK’s atomic force microscope to the Genomics Resource Center and High-Performance Computing Cluster at Rockefeller University.
Prescott commented, “This is a great example of how investment in ‘basic science’ and investigation of fundamental biological questions can open the door to medical advances,” he said. “I always thought I’d be working on questions that decades later someone might cite in a paper when they come up with a cure for some disease. Never in a million years did I expect to lead a project that identified such a strong candidate for drug development for a global scourge like hepatitis B.”